Het arrangement Introduction to the Biobased Economy is gemaakt met Wikiwijs van Kennisnet. Wikiwijs is hét onderwijsplatform waar je leermiddelen zoekt, maakt en deelt.
- Auteur
- Laatst gewijzigd
- 2018-12-28 13:10:57
- Licentie
-
Dit lesmateriaal is gepubliceerd onder de Creative Commons Naamsvermelding 3.0 Nederlands licentie. Dit houdt in dat je onder de voorwaarde van naamsvermelding vrij bent om:
- het werk te delen - te kopiëren, te verspreiden en door te geven via elk medium of bestandsformaat
- het werk te bewerken - te remixen, te veranderen en afgeleide werken te maken
- voor alle doeleinden, inclusief commerciële doeleinden.
Meer informatie over de CC Naamsvermelding 3.0 Nederland licentie.
Project informatie en samenwerkingsverbanden
Dit project is tot stand gekomen door een samenwerking binnen het Centre of Biobased Economy (getrokken door Wageningen UR, HAS Hogeschool, INHolland Delft, VanHaLL Larenstein en CAH Vilentum). Om de ontwikkeling naar een sterke biobased economy verder door te zetten bundelen onderwijs en bedrijfsleven hun krachten binnen het Centre fo Biobased Economy (CBBE) om professionals op te leiden. Het CBBE doet dat door:
- Kennisoverdracht en onderwijsontwikkeling
- Versterking toepassingsgericht onderzoek
- Stimulering van innovatieprojecten
Dit initiatief is mede mogelijk gemaakt door het ministerie van Economische Zaken.
Het doel van deze wikiwijs module is om een zo groot mogelijk aantal studenten te bereiken.
Meer informatie
Deze oplage is voor een ieder openbaar te gebruiken. U mag het Document kopiëren en verspreiden in elk medium, alleen voor niet-commerciële doeleinden, onder het voorbehoud dat deze Licentie, de auteursrechtelijke kennisgevingen en de licentiekennisgeving die aangeeft dat deze Licentie van toepassing is op het Document weergegeven worden in alle kopieën, en dat u geen enkele andere voorwaarde toevoegt aan de voorwaarden van deze Licentie. U mag geen technische maatregelen nemen die het lezen of verder kopiëren van de exemplaren die u maakt of verspreidt belemmeren of controleren.
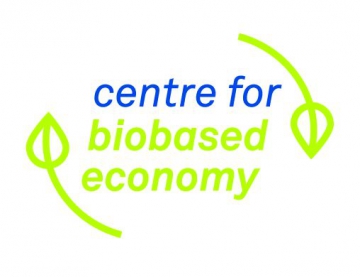
Aanvullende informatie over dit lesmateriaal
Van dit lesmateriaal is de volgende aanvullende informatie beschikbaar:
- Leerniveau
- HBO - Bachelor;
- Leerinhoud en doelen
- Economie; Procestechnologie;
- Eindgebruiker
- leerling/student
- Moeilijkheidsgraad
- gemiddeld
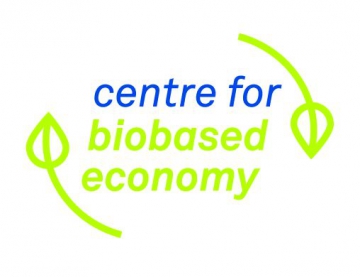
Bronnen
Bron | Type |
---|---|
Centre of Biobased Economy http://www.cbbe.nl |
Link |
First introduction video https://youtu.be/XX6911pS0Kg |
Video |
Second introduction video https://youtu.be/2xvXkOMRTs4 |
Video |
Circulair Economy... it's the way forward https://www.youtube.com/watch?v=lK00v_tzkCI |
Video |
Global warming https://www.youtube.com/watch?v=G4H1N_yXBiA |
Video |
Why is COP24 So Important? - Conference on Climate Change https://www.youtube.com/watch?v=xKBtWXJfnjE |
Video |
Fuel from agricultural residues https://youtu.be/LAoy0kwK7e0 |
Video |
DSM-POET and local economical development in Iowa https://youtu.be/GlaAoGDQNPk |
Video |
https://vimeo.com/blocnl/biobasedbackbone https://vimeo.com/blocnl/biobasedbackbone |
Link |
https://www.agro-chemistry.com/ https://www.agro-chemistry.com/ |
Link |
https://youtu.be/zCRKvDyyHmI https://youtu.be/zCRKvDyyHmI |
Video |
https://www.ellenmacarthurfoundation.org/circular-economy/infographic https://www.ellenmacarthurfoundation.org/circular-economy/infographic |
Link |
https://www.youtube.com/watch?v=1af08PSlaIs https://www.youtube.com/watch?v=1af08PSlaIs |
Video |
https://www.youtube.com/watch?v=Oovu6oeFlw8 https://www.youtube.com/watch?v=Oovu6oeFlw8 |
Video |
Hemp production and valorisation: which products can be made from hemp? https://youtu.be/m-7wrT1Upvw |
Video |
https://www.youtube.com/watch?v=nyEu2KJRBeY https://www.youtube.com/watch?v=nyEu2KJRBeY |
Video |
https://www.youtube.com/watch?v=CQBw9JsBnI4 https://www.youtube.com/watch?v=CQBw9JsBnI4 |
Video |
https://www.youtube.com/watch?v=igUtLwruUjA https://www.youtube.com/watch?v=igUtLwruUjA |
Video |
https://maken.wikiwijs.nl/bestanden/250288/Opdracht%20business%20case%20HarvestaGG.docx https://maken.wikiwijs.nl/bestanden/250288/Opdracht%20business%20case%20HarvestaGG.docx |
Link |
Explanation of photosynthesis https://youtu.be/g78utcLQrJ4 |
Video |
https://www.youtube.com/watch?v=KUApt4RIU1M https://www.youtube.com/watch?v=KUApt4RIU1M |
Video |
Haber-Bosch process https://www.youtube.com/watch?v=VtQFiwHlnmY |
Video |
Fosfaat: tekort aan wat nooit opraakt? https://www.youtube.com/watch?v=HqOfcUufyzQ |
Link |
Biomassa: wensen en grenzen http://infographics.pbl.nl/biomassa/# |
Link |
Routekaart https://www.biobasedeconomy.nl/routekaart/ |
Link |
Explanation of the structures of the basic biomolecules https://youtu.be/PYH63o10iTE |
Video |
https://www.youtube.com/watch?v=qht5D8Hn5d8 https://www.youtube.com/watch?v=qht5D8Hn5d8 |
Video |
https://www.youtube.com/watch?v=hzZTISz4Rgw https://www.youtube.com/watch?v=hzZTISz4Rgw |
Video |
Manure, a valuable resource http://edepot.wur.nl/294017 |
Link |
https://youtu.be/niZls2dpHjM https://youtu.be/niZls2dpHjM |
Video |
https://www.youtube.com/watch?v=ieuW8GUdTSM https://www.youtube.com/watch?v=ieuW8GUdTSM |
Video |
https://www.youtube.com/watch?v=KtUWDzAsR-w https://www.youtube.com/watch?v=KtUWDzAsR-w |
Video |
https://www.youtube.com/watch?v=TRNIvoIlJ-Q https://www.youtube.com/watch?v=TRNIvoIlJ-Q |
Video |
https://youtu.be/MDmW2BKaSPY https://youtu.be/MDmW2BKaSPY |
Video |
https://www.dsm.com/corporate/about/business-entities/dsm-biobased-productsandservices.html https://www.dsm.com/corporate/about/business-entities/dsm-biobased-productsandservices.html |
Link |
http://www.corbion.com/biochemicals/co-creation-makes-us-greater-together http://www.corbion.com/biochemicals/co-creation-makes-us-greater-together |
Link |
https://www.youtube.com/watch?v=0k-7_VhR38U https://www.youtube.com/watch?v=0k-7_VhR38U |
Link |
https://www.arkema.com/en/markets-and-solutions/solutions/composites-solutions/ https://www.arkema.com/en/markets-and-solutions/solutions/composites-solutions/ |
Link |
https://www.youtube.com/watch?v=x243jvUCaaM https://www.youtube.com/watch?v=x243jvUCaaM |
Link |
https://www.youtube.com/watch?v=2XsYvS4Os5M https://www.youtube.com/watch?v=2XsYvS4Os5M |
Link |
https://www.youtube.com/watch?v=fIbLHiTZaYM https://www.youtube.com/watch?v=fIbLHiTZaYM |
Link |
https://www.foodvalleyupdate.com/food-valley-nl/papaerfoam-sustainable-design-for-champagne-packaging/ https://www.foodvalleyupdate.com/food-valley-nl/papaerfoam-sustainable-design-for-champagne-packaging/ |
Link |
Proeffabriek voor bioplastic uit gft in Venlo http://www.technischweekblad.nl/de-ultieme-kringloop.345076.lynkx |
Link |
Torrefactie: biomassa omzetten in groene steenkool http://edepot.wur.nl/188892 |
Link |
Innovatieprijs Symbra Biofoam https://youtu.be/o8VcdKHU-yU |
Video |
https://nl.wikipedia.org/wiki/Business_Model_Canvas https://nl.wikipedia.org/wiki/Business_Model_Canvas |
Link |
The Biobased Initiative Joint Undertaking https://youtu.be/-lYj_AIjEWs |
Video |
Greenwashing: False Claims on Recycling, Sustainability and Eco Friendly Products https://youtu.be/1QUKuA4Obcw |
Video |
Are Aluminium Water Bottles better? https://youtu.be/UX56b9BmV80 |
Video |
Strategie voor een groene samenleving, Biomaterialen drijfveer voor de biobased economy http://http://www.biobasedeconomy.nl/wp-content/uploads/2013/12/5803-Visie-op-WTC-binnenwerk-WEB.pdf |
Link |
Naar groene chemie en groene materialen, WTC BBE http://edepot.wur.nl/165546 |
Link |